- HCC-78
- HDLM-2
- DOHH-2
- L-540
- MX-1
- NALM-6
- NB-4
- CAL-51
- SNB-19
- KYSE-520
- MKN-45
- BA/F3
- MS-5
- HCEC-B4G12
- NK-92
- PA-TU-8988S
- MONO-MAC-1
- PA-TU-8902
- Human Microglia
- Human Hepatic Stellate Cells
- Human Skeletal Muscle Cells (DMD)
- Human Schwann Cells
- Human Oral Keratinocytes (HOK)
- Human Cardiomyocytes
- Human Small Intestinal Epithelial Cells
- Human Colonic Epithelial Cells
- Human Intestinal Fibroblasts
- Primary Human Large Intestine Microvascular Endothelial Cells
- Human Small Intestinal Microvascular Endothelial Cells
- Human Retinal Pigment Epithelial Cells
- Human Hepatocytes
- Cynomolgus Monkey Lung Microvascular Endothelial Cells
- Cynomolgus Monkey Vein Endothelial Cells
- C57BL/6 Mouse Primary Mammary Epithelial Cells
- C57BL/6 Mouse Vein Endothelial Cells
- Rat Primary Kidney Epithelial Cells
- Rat Gingival Epithelial Cells
- Rabbit Lung Endothelial Cells
Our Promise to You
Guaranteed product quality, expert customer support
ONLINE INQUIRY
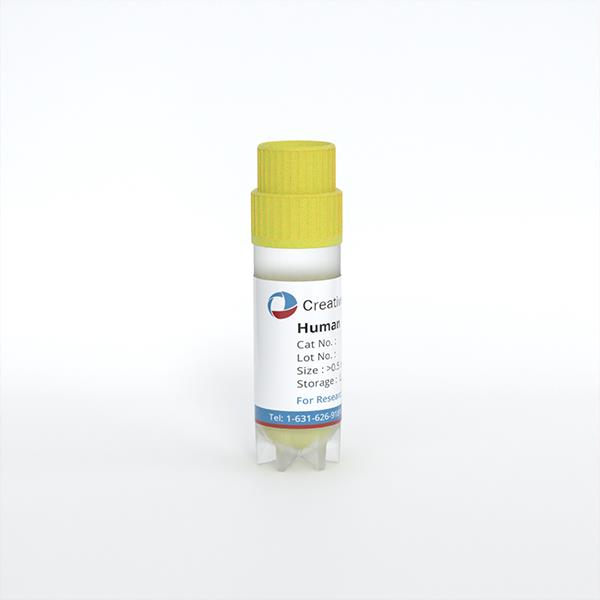
Human Liver Carcinoma Epithelial Cells
Cat.No.: CSC-C9218J
Species: Human
Source: Liver
Cell Type: Epithelial
- Specification
- Background
- Scientific Data
- Q & A
- Customer Review
Hepatocellular carcinoma (HCC) is the most common type of primary liver cancer and is generally associated with various factors, including HBV, HCV, liver cirrhosis, excessive alcohol consumption, chemical carcinogens like aflatoxins, and environmental factors. Human liver carcinoma epithelial cells, also known as hepatocellular carcinoma (HCC) cells, originate from liver cancer tissues and possess tumor cell line characteristics with adhering growth. These cells typically exhibit epithelial morphologies such as polygonal, round, or oval shapes. They also perform a variety of differentiated liver functions, including plasma protein synthesis and secretion, cholesterol and triglyceride metabolism, lipoprotein metabolism and transport, bile acid synthesis, glycogen synthesis, and insulin signaling.
Compared to primary hepatocytes, this cell line offers several advantages, including an unlimited lifespan, stable phenotype, high availability, and ease of handling. Additionally, it is derived from the pathological liver tissue of diagnosed HCC patients, showing genetic similarities to those patients. Its development mechanisms, functional protein expression, invasion and metastasis capabilities, and drug resistance closely resemble those of HCC patient cells. Furthermore, its drug resistance profile mirrors that of HCC patient cells, making it an invaluable tool for evaluating the efficacy and toxicity of potential therapeutic agents. Therefore, it is commonly used as a substitute for primary hepatocytes in drug metabolism and hepatotoxicity studies. It provides a reliable model for understanding the disease mechanisms at a genetic and cellular level, facilitating the development and testing of new therapeutic interventions. However, it has certain limitations, such as lower expression of some metabolic activities compared to hepatocytes, necessitating careful consideration based on specific experimental needs.
Fig. 1. Human liver carcinoma epithelial cells (HepG2) (Donato, M. T., Tolosa, L., et al., 2015).
A HepG2 Cell-Based Biosensor That Uses Stainless Steel Electrodes for Hepatotoxin Detection
Environmental hepatotoxins can lead to liver failure. Biosensors may be the optimal candidates for detecting hepatotoxins. Live cells can serve as recognition elements in biosensors, but they may have difficulty adhering to the surface. This study investigated the suitability of HepG2 (hepatocellular carcinoma epithelial cells) as working electrodes in biosensors, assessing their adhesion capability on stainless steel surfaces. The sensor's performance was validated by detecting the viability of HepG2 cells exposed to different hepatotoxins.
Both the neutral red and coomassie brilliant blue cytotoxicity assays showed that cell viability decreased with higher concentrations of hepatotoxins (aflatoxin B1, AFB1 and isoniazid, INH). This indicates that both compounds exhibit hepatotoxic responses and can help assess the biosensor's limit of detection (LOD). Optical microscopy revealed a gradual decrease in HepG2 cell coverage with increasing AFB1 and INH concentrations, suggesting reduced tissue structure affinity. At higher concentrations, HepG2 cells underwent necrosis (Fig. 1). Fluorescence microscopy confirmed that in the HepG2 control group, many live, adherent cells were observed. As AFB1 concentration increased, live cell numbers decreased while dead cells increased, aligning with AFB1's hepatotoxic effects. For INH, dead cell numbers increased at 5 and 50 mM but were lower at 250 mM, possibly due to cell detachment or complete necrosis at high concentration. (Fig. 1b and c).
Electrochemical impedance spectroscopy (EIS) measurements were conducted to evaluate the efficacy of HepG2 cells adhered to a stainless steel surface as a biosensing device. For AFB1 assays, cells were almost completely shed. The Bode plot showed minimal overall impedance change, with significant phase differences below 1 Hz between the untreated control and treated biosensor, indicating no cell attachment (Fig. 2a and c). For INH testing, the difference between negative and positive controls was smaller, with slightly lower impedance due to potential increased conductivity from metabolites or necrosis products. Similar to AFB1, minimal impedance change was observed with slight differences among controls and samples (Fig. 2b and d). These results suggest that this biosensor can detect the presence of different hepatotoxins.
Fig. 1. Optical microscopy photographs under the influence of AFB1 on the HepG2 cell line (a) and impact of AFB1 and INH on HepG2 cell survival (b-c) (Rozman M., Štukovnik Z., et al., 2022).
Fig. 2. Nyquist diagrams of investigated biosensors showing a response for aflatoxin B1 (A) and isoniazid (B), along with Bode diagrams for aflatoxin B1 (C) and isoniazid (D) (Rozman M., Štukovnik Z., et al., 2022).
Lactonic Sophorolipid–Induced Apoptosis in Human HepG2 Cells through the Caspase-3 Pathway
Sophorolipids (SLs) are biosurfactants produced by non-pathogenic yeast and secondary metabolites secreted by microorganisms under certain culture conditions. They are mainly classified into lactonic sophorolipids (LSL) and acidic sophorolipids. Among these, LSL possess significant biological activities such as antibacterial, antiviral, and anti-inflammatory effects, making them potential candidates as anti-cancer agents. Consequently, Wang's team explore the impact of LSL on the proliferation of human liver carcinoma epithelial cells (HepG2 cells) in vitro. The results showed that LSL may inhibit the growth of HCC cells by inducing apoptosis.
To investigate the molecular mechanism of LSL-induced apoptosis, they examined the enzyme activity of Caspase-3 and Caspase-9 in each group. They found that in LSL and etoposide intervention groups, the expression of Caspase-3 and Caspase-9 enzyme activity increased (Fig. 3a and b). This suggested that the apoptosis induced by LSL may depend on the Caspase pathway in HepG2 cells. In order to understand the molecular mechanism underlying the apoptosis induced by LSL, the apoptosis-related genes in HepG2 cells treated with LSL and etoposide were measured. The expression of Apaf-1, Caspase-3, and Bax mRNA in each group increased, and the expression of Bcl-2 mRNA decreased (Fig. 3c-f). To get to know how it functioned, they measured the expression of apoptosis-related proteins. The experimental results identified that the expression of Caspase-3, Cleaved Caspase-3, Bcl-2, and Bax proteins in each group increased after the intervention of LSL and etoposide. The expression of Bcl-2 protein was reduced (Fig. 4). In conclusion, these results displayed that LSL changed related apoptosis genes and proteins in liver cancer cells, which further showed that LSL can induce apoptosis in liver cancer cells.
Fig. 3. Activity detection of Caspase-3 and Caspase-9 enzyme activity (a-b) and expression of related genes (c-f) after LSL and etoposide intervention in HepG2 cells (Wang, X., Xu, N., et al., 2021).
Fig. 4. Expression of related proteins after LSL and etoposide intervention in HepG2 cells (Wang, X., Xu, N., et al., 2021).
Ask a Question
Write your own review