ONLINE INQUIRY
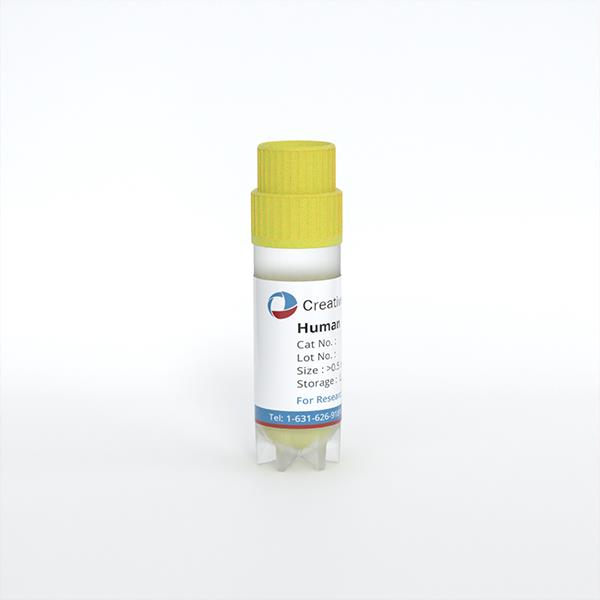
Human Epidermal Keratinocytes-neonatal (HEK-n)
Cat.No.: CSC-7789W
Species: Human
Source: Epidermis; Skin
Cell Type: Keratinocyte
- Specification
- Background
- Scientific Data
- Q & A
- Customer Review
Human epidermal keratinocytes-neonatal (HEK-n), derived from the epidermis of newborns, serve as the primary protective barrier of the skin. They typically present as flattened or polygonal cells, proliferating continually from the basal cells. These cells produce robust keratin while migrating upwards, gradually forming the stratum corneum, and form tight intercellular connections with structures like desmosomes, thereby preventing water loss and blocking harmful external substances to maintain skin integrity and barrier function. When the skin is damaged, these cells rapidly proliferate and migrate to partake in skin repair and regeneration processes. Concurrently, they secrete various cytokines to modulate T lymphocyte activity, participating in the skin's immune response and inflammatory processes.
To accommodate the rapid growth demands of neonates, HEK-n exhibit high proliferative activity. By integrating with other cellular components and biomaterials, they can be engineered into skin tissues mimicking the structure and function of natural skin, serving in the treatment and repair of large-scale skin injuries like burns and wounds. Furthermore, as crucial models for testing dermatological drugs and cosmetics, HEK-n can simulate real human skin to evaluate the permeability, safety, and efficacy of these products. Researching the signaling pathways related to differentiation, proliferation, and immune function in these cells allows scientists to unravel complex biological processes associated with skin health and diseases.
Fig. 1. Undifferentiated neonatal human epidermal keratinocytes (Sehgal N, Seifert B, et al., 2016).
Oleic Acid Enhances Keratinocytes Differentiation Via the Upregulation of miR-203 in Human Epidermal Keratinocytes
FFAs modulate keratinocyte differentiation. Oleic acid (OA) is an unsaturated free fatty acid (FFA) constituent of sebum. Kim's team examined changes in intracellular protein and mRNA expression levels after treating neonatal human epidermal keratinocytes (HEKn) with different concentrations of OA, while using immunofluorescence staining to study cytoskeletal changes. To explore whether OA affects keratinocyte differentiation in HEKn.
The viability of HEKn cells treated for 24 hours OA at concentrations below 5 μmol/L remained over 80% (Fig. 1A and B). After 3 days of OA treatment, the mRNA (Fig. 1C and D) and protein levels (Fig. 1E) of keratin 10 and involucrin increased in a dose-dependent manner. Correspondingly, the number of keratin 10- and involucrin-positive HEKn also rose (Fig. 1F), indicating that OA promotes keratinocyte differentiation. The OA-treated HEKn colonies showed stratification (birefringent light scattering) after 3 days (Fig. 1G). OA prompted cytoskeletal changes, including actin ring organization, loss of planar polarity, and increased E-cadherin at cell contacts (Fig. 2A). miR-203 triggers keratinocyte differentiation by repressing the expression of p63. They found that OA treatment also elevated miR-203 levels, reducing p63 expression (Fig. 2B and C). They then determined whether OA-induced keratinocyte differentiation is associated with miR-203 upregulation. Transfection with anti-miR-203 inhibited the OA-driven miR-203 expression and mitigated the decrease in p63 and involucrin expression but not keratin 10 (Fig. 2D and E). The above results demonstrates that treatment with OA accelerates keratinocyte differentiation via the upregulation of miR-203 in HEKn under sub-confluent conditions, suggesting that OA in the sebum is involved in the development and maturation of the skin, especially the epidermis
Fig. 1. Oleic acid (OA) promotes the differentiation of human keratinocytes (Kim YJ, Lee SB, et al., 2019).
Fig. 2. Oleic acid (OA) accelerates the differentiation of neonatal human epidermal keratinocytes (HEKn) via the upregulation of miR-203 (Kim YJ, Lee SB, et al., 2019).
CLSP Activates htHNR and STAT3 Signaling to Suppress Senescence of NHEKs
Calmodulin-like skin protein (CLSP) is a secreted peptide produced by skin keratinocytes and some related epithelial cells. In the central nervous system, it combats Alzheimer's disease (AD) by inhibiting neuronal cell death through receptor binding, a process mediated by the heterotrimeric humanin receptor (htHNR). Consequently, CLSP is proposed to be a primary agonist for htHNR, whose messenger RNA (mRNA) for three subunits is likely expressed in skin tissues. Thus, CLSP secreted by skin keratinocytes may exhibit certain biological activities in skin tissue. Using neonatal human epidermal keratinocytes (NHEKs) treated with hydrogen peroxide (H2O2) or exposed to ultraviolet (UV) irradiation as models for keratinocyte senescence, Takahara's team investigated whether CLSP affects keratinocyte aging and its mechanism.
Takahara's team first examined the expression of CLSP in the aging model, and the results showed that CLSP expression was up-regulated. They hypothesized that CLSP expression is upregulated to counteract cell senescence. To test this, recombinant CLSP was added to cultured NHEKs with artificially induced senescence. Recombinant CLSP, produced using a bacterial system (Fig. 3A), significantly reduced the H2O2-induced increase in senescence-associated (SA) β-galactosidase-positive cells (Fig. 3B and 3C). Similarly, it reduced the UV-B-induced senescence (Fig. 3D). However, CLSP-ΔN2, which lacks the crucial humanin-homologous domain, did not significantly reduce UV-B-induced senescence (Fig. 3D). The reduction in senescence by CLSP is partial, possibly due to the rapid senescence in models or the need for cooperation with other factors. SA β-galactosidase activity was used as a senescence marker, while nonspecific markers like cyclin-dependent kinase inhibitors, p16Ink4A, and p21Cip1, were also considered. In these models, p16Ink4A was not induced by H2O2 (Fig. 4), but p21Cip1 was, and CLSP did not reduce the increase in p21Cip1 (Fig. 4). Their interpretation is that H2O2 induces both cell-cycle arrest and senescence via oxidative stress, while CLSP affects only senescence. The above results show that CLSP activates intracellular signaling via htHNR and STAT3, influencing gene transcription to suppress senescence.
Fig. 3. CLSP reduces the H2O2-induced increase or the UV-B-induced increase in senescence-associated (SA) β-galactosidase activity (Takahara Y, Miyachi N, et al., 2019).
Fig. 4. Treatment with H2O2 induced the expression of p21cip1 but, not of p16Ink4A (Takahara Y, Miyachi N, et al., 2019).
Ask a Question
Write your own review